Upper Midwest Environmental Sciences Center
Species Distributional Modeling and Spatial Patterns in Genetic Introgression: the Case of Golden-winged Warblers and Blue-winged Warblers
Abstract
Using models and maps of species distribution, we predicted the extent of population overlap between two similar Vermivora species, the Golden-winged Warbler (V. chrysoptera) and the Blue-winged Warbler (V. pinus) in the Prairie Hardwood Transition zone of the upper midwestern United States. We found that Blue-winged Warblers were, in general, occurring at three times the rate of Golden-winged Warblers in the region. The ratio of overlap was greater in Wisconsin (8.4 Blue-winged Warblers : 1 Golden-winged Warbler) than Minnesota (4.4:1), which was roughly coincident with reported patterns in introgression between these species (10% and 1% introgression, respectively). If introgression is a function of population density, our results suggested levels of introgression in Minnesota would likely increase in time to ratios similar to that observed in Wisconsin. We surmise that conserving populations of Golden-winged Warblers free of introgression in the Prairie Hardwood Transition may not be possible for any great length of time given the spatial patterns in abundance of Blue-winged Warblers. Thus, we recommend conjoint investigations into spatial patterns in introgression and species distribution north of the Prairie Hardwood Transition where the global core of the Golden-winged Warbler population occurs.
Introduction
The Golden-winged Warbler (Vermivora chrysoptera) is a species of considerable conservation concern in North America, primarily due to widespread population declines attributable to habitat loss (Confer et al. 1992, Buehler et al. ) and displacement by the Blue-winged Warbler (V. pinus) in areas of sympatry (Gill 1980, 2004; Will 1986). Recent research highlighted an important mechanism in the interactions of these two species, genetic introgression resulting in hybridization (Shapiro et al. 2004, Vallender et al. 2007a, b; 2009). Vallender et al. (2009) reported 8% of sampled Golden-winged Warblers were genetically introgressed. Their major conclusion was that the only population remaining free of introgression was in Manitoba (and possibly Ontario), Canada.
Conserving an evolutionarily significant unit requires the maintenance of populations free from introgression (Rhymer and Simberloff 1996); as geographic separation between these genetically similar species abates, the task of conserving their unique evolutionary heritage grows more difficult. Vallender et al. (2009) suggested that to protect populations of Golden-winged Warblers from genetic introgression with Blue-winged Warblers will require identifying the scale and specific locations at which genetically pure breeding populations exist. At a fundamental level, such a task requires an understanding of a species’ distribution.
Species distributional modeling is a major facet of conservation biogeography (Fitzgerald et al. 2008, Thogmartin et al. 2004, 2007, 2009) and plays an important role in the U.S. Fish and Wildlife Service’s business of Strategic Habitat Conservation (Johnson et al. 2008). Results from this type of correlative ecological niche modeling have provided valuable insight into many areas of conservation and evolutionary biology, including historical and future patterns in biogeography (Peterson et al. 2002, Martinez-Meyer et al. 2004, Araújo et al. 2006, Wiens et al. 2006), niche conservatism (Peterson et al. 1999, Stephens and Wiens 2009), regions of high endemicity (Rissler et al., 2006), and invasion ecology (Peterson and Robins 2003).
We suggest that species distributional models can provide additional insight into the degree and spatial patterning of distributional overlap we may expect between conspecific organisms. From these patterns in distributional overlap, we can hypothesize the 1) spatial extent of populations at risk to genetic introgression, and 2) areas which may remain free of substantive introgression. We elaborate briefly on each of these avenues of insight available through species distributional modeling, using the case of Blue-winged Warbler and Golden-winged Warbler populations in the midwestern United States.
Methods
Study Area
We modeled patterns in species abundance for the populations occurring in Bird Conservation Region 23, the Prairie Hardwood Transition (U.S. NABCI Committee 2000). The Prairie Hardwood Transition occupies 230,111 km2, stretching from central Minnesota through central and southern Wisconsin and Michigan, including small sections of northeastern Iowa, and northern Illinois and Indiana; Lake Michigan bisects the region. The predominant land uses/land covers in this region are row crop agriculture (36%), agricultural grassland (27%), and deciduous forest (21%), of which Aspen/Birch (Betula spp.) comprised approximately half that amount.
Modeling Approach
We developed species distributional models for both the Blue-winged Warbler (LeBrun, Thogmartin, and Miller, unpublished data) and Golden-winged Warbler (Thogmartin, unpublished data) in BCR23. Because these data and models are, as of this date, unpublished, we briefly recount the methods we used to map patterns in the occurrence and abundance of these species.
We used 1840 bird counts collected by the North American Breeding Bird Survey (BBS) as the response in our models (Thogmartin et al. 2004). Each BBS route contains 50 evenly-spaced survey locations (stops) at which an observer counts all birds seen or heard in a 3-min period. We used the sum of counts from the 50 stops in a year’s route survey as an index of abundance along the route for that year. The 1840 counts we used for model building were produced by 310 observers over 140 routes between 1981 and 2001; an additional 396 counts were held back for model evaluation. Over a 20-year period observers changed; not all routes were run each year and no route was run more than once per year.
Bird counts from the BBS were similar to each other to varying degrees because of temporal and spatial correlation and correlated observational error (Link and Sauer 2002, Thogmartin et al. 2004), each of which created a level of correlated structure between survey counts. In combination with the other random effects, the nesting of observers within routes over time constituted the statistical hierarchy organizing the data. Therefore, we employed a hierarchical modeling approach consisting of fixed and random effects to map predicted abundances at a regional scale. Thogmartin et al. (2004; elaborated further in Thogmartin et al. 2006, 2007) introduced the methodology we employed to derive associations between bird abundance and environmental variables. Counts were modeled as a loglinear function of a priori-identified explanatory variables describing habitat, spatial relatedness, and individual effects of observer and year. Land cover composition was derived from the National Land Cover Dataset 1992 (Vogelmann et al. 2001) and the Forest Resources of the United States Forest Cover Type Dataset 1997 (Zhu and Evans 1994); unfortunately, mapped forest structural information was not available. Spatial dependence between survey counts was incorporated as a first-order conditional autoregression based upon an irregular lattice describing the spatial neighborhood of routes (Thogmartin et al. 2004).
We conducted model fitting in WinBUGS (Spiegelhalter et al. 2003), a statistical package conducting Bayesian inference with Markov chain Monte Carlo methods (Gibbs Sampling) (Link and Sauer 2002). For each model we ran the Markov chain until convergence occurred (>15,000 iterations) and then an additional 3,000 iterations past convergence. This chain creation was conducted 3-5 times to create replicate chains for the Gelman-Rubin diagnostic (Brooks and Gelman 1998, Spiegelhalter et al. 2003), comparing within-chain and between-chain variability. Code similar to the model we implemented is available in Thogmartin et al. (2004).
We mapped the final statistical model in the geographic information systems ArcGIS 9.2 (Environmental Systems Research, Inc., Redlands, California, USA) by combining grid layers based upon their model-averaged slope coefficients. Details of the mapping process are described more fully in Thogmartin et al. (2004, 2006).
Characterizing the Tension Zone
Species differ in their detectability. A fundamental difficulty in using Breeding Bird Survey data is that this survey does not explicitly control for these potential differences in species detectability. Partners in Flight amended Breeding Bird Survey counts by various adjustment factors to overcome this issue of species detectability when calculating regional and continental population sizes for landbirds (Rich et al. 2004, Rosenberg and Blancher 2005); the only factor they suggested that differed between Golden-winged Warblers and Blue-winged Warblers was a time-of-day (ToD) adjustment (ToDGWWA = 1.32, ToDBWWA = 1.21). In locations where the same mean BBS count is observed, Golden-winged Warblers are predicted to be ~8% more abundant simply because of differences in detectability. Thus, if we take the Partners in Flight method as true, Golden-winged Warblers are approximately 31% as abundant as Blue-winged Warblers. However, because of the great scrutiny that these species have received in recent years (Kubel and Yahner 2007, Confer et al. 2008), these two species may also differ in the distance over which they can be detected. Thus, because of these difficulties in amending Breeding Bird Survey counts to reflect true abundance, we constrained our analyses to patterns in relative abundance rather than estimated population size. This use of relative abundance may not be a major drawback if introgression and hybridization occur as a function of relative rather than absolute population size. To identify areas of overlap between these species, we mapped the ratio of Blue-winged Warblers to Golden-winged Warblers, hypothesizing that introgression should be greatest in areas where this ratio was greatest.
Results
Golden-winged Warblers are nearly absent from lower Michigan in BCR23 (Fig. 1a). The largest areas of predicted abundance occurred in west-central Wisconsin and along the border between BCR23 and Bird Conservation Region 12 (BCR12), the Boreal Hardwood Transition. Our predictions of Blue-winged Warbler indicated that this species was found virtually throughout BCR23; there appears, however, to be an east to west gradient in the occurrence and abundance of this species, with Blue-winged Warblers being most highly abundant in lower Michigan and least abundant in Minnesota (Fig. 1b).
Areas of overlap between these species, where Blue-winged Warblers were most abundant relative to Golden-winged Warblers, indicated patterns that were very similar to the prediction surface for Blue-winged Warblers (Fig. 2). However, areas of southwestern Michigan, where overlap was low, were a consequence of very few Golden-winged Warblers being predicted for that portion of the state. Conversely, in Minnesota, where Blue-winged Warblers were in very low abundance, intensity of overlap was also predicted to be low. In central Wisconsin, where Golden-winged Warblers were still abundant, the intensity of overlap was predicted to be low, though this intensity increased as one moved westward.
Discussion
The Golden-winged Warbler risks extinction through hybridization and introgression with the Blue-winged Warbler (Rhymer and Simberloff 1996, Crandall et al. 2000). However, before this risk of extinction through genetic processes may take place, the Golden-winged Warbler faces potentially more immediate risk of local extirpation through aggressive interactions with its sister species. The model Thogmartin (unpublished data) used for predicting patterns in abundance for Golden-winged Warblers included a covariate for Blue-winged Warblers (βBWWA = -0.33); the magnitude and direction of this estimate indicated a decline in abundance of one-third of a Golden-winged Warbler for every 1 bird increase in Blue-winged Warblers. As we noted earlier, Golden-winged Warblers are at a mean abundance of ~1/3 that of Blue-winged Warblers in BCR23 (assuming issues of detection do not confound this ratio too greatly). This suggests that, if behavioral interaction between these species is a factor in the decline of Golden-winged Warbler populations, Blue-winged Warblers would appear to be sufficiently abundant to push the entire Golden-winged Warbler population from the region. Golden-winged Warblers apparently persist to this point in the region simply through a partitioning of the regional space.
Will (1986) described behavioral interactions between Golden-winged Warblers and Blue-winged Warblers in Michigan, at a time when Blue-winged Warblers were relatively new to lower Michigan. Since Will’s studies in the early- to mid-1980s, Golden-winged Warblers have retreated further from lower Michigan in the face of an increasing Blue-winged Warbler population (Fig. 1a). Today, the contest between these two species is in the Wisconsin portion of BCR23. The southern edge of the most abundant population of Golden-winged Warblers is fronted with a zone of high predicted overlap between species. It is in this area where it appears Blue-winged Warblers have the greatest potential for eliminating Golden-winged Warblers from the region, should they continue to move northward in a manner akin to what occurred in Michigan in the 1980s and 1990s.
Conservation Implications
We propose that there are still likely to be populations in BCR23 largely unaffected by substantial introgression, but these are few in number and extent, and probably in decline. Vallender et al. (2009) reported that sampled Golden-winged Warblers (which undoubtedly included samples from BCR12) in Wisconsin exhibited a rate of introgression of nearly 10% (n = 12 introgressed individuals out of 123 total birds sampled), whereas in Minnesota, the rate of introgression was only 1% (n = 1/96). This pattern in introgression is reflected in the distributional predictions for these species, where remaining areas of high Golden-winged Warbler abundance in Minnesota are predicted to exhibit low levels of overlap with Blue-winged Warblers (Table 1). However, because of spatial patterns in the predicted overlap, we expect introgression in the Minnesota portion of the population to increase at least 5-fold as the levels of introgression equilibrate to levels of overlap in population abundance. Should the population of Blue-winged Warblers continue to increase and extend their north- by northwest range expansion, the level of introgression in Minnesota will likely increase as well. Therefore, conserving populations of Golden-winged Warblers free of introgression in BCR23 may not be possible for any great length of time given the spatial patterns in abundance of Blue-winged Warblers. As this species moves into areas formerly occupied by Golden-winged Warblers, Golden-winged Warblers often persist in small local populations in moister portions of the forested landscape (Will 1986). But this persistence also occurs at increasing levels of intermixing with the Blue-winged Warbler. Our current understanding of species-habitat relations provide us too little guidance for how to manage these two species in areas of sympatry for minimizing behavioral interaction.
Unlike these two Vermivora species, there is relatively little interaction between population geneticists and avian biogeographers. We recommend an increased degree of collaboration in developing conservation strategies which are cognizant of habitat-specific patterns in behavioral and reproductive interaction over potentially broad spatial scales (Manel et al. 2003, Scribner et al. 2005, Storfer et al. 2007). Conjoint spatial and temporal models of introgression and species distribution will likely help identify areas and strategies for maintaining species integrity. We recommend these studies be conducted in the Boreal Hardwood Transition where the Golden-winged Warbler maintains the core of its range.
Acknowledgements
We appreciate the considerable investment of time and effort by the numerous individuals associated with the North American Breeding Bird Survey, without which this study would not have been possible.
Literature cited
Araújo, M. B., W. Thuiller, and R. G. Pearson. 2006. Climate warming and the decline of amphibians and reptiles in Europe. Journal of Biogeography 33:1712–1728.
Buehler, D. A., A. M. Roth, R. Vallender, T. C. Will, J. L. Confer, R. A. Canterbury, S. Barker Swarthout, K. V. Rosenberg, and L. P. Bulluck. 2007. Status and conservation priorities of golden-winged warblers (Vermivora chrysoptera) in North America. The Auk 124:1439–1445.
Confer, J. L. 1992. Golden-winged Warbler (Vermivora chrysoptera) In A. Poole, P. Stettenheim and F. Gill, editors. Birds of North America. Number 20. Academy of Natural Sciences, Philadelphia, Pennsylvania, USA, and American Ornithologists’ Union, Washington, D.C., USA.
Confer, J. L., R. E. Serrell, M. Hager, and E. Lahr. 2008. Field tests of the Rosenberg-Blancher method for converting point counts to abundance estimates. The Auk 125:932–938.
Crandall et al. 2000. Considering evolutionary processes in conservation biology. Trends in Ecology and Evolution 15:290-295.
Fitzgerald, J. A., W. E. Thogmartin, R. Dettmers, T. Jones, C. Rustay, J. M. Ruth, T. C. Will, and F. R. Thompson III. 2008. Application of models to conservation planning for terrestrial birds in North America. Pages 593–624 in J. J. Millspaugh and F. R. Thompson III, editors. Models for planning wildlife conservation in large landscapes. Academic/Elsevier, Boston, Massachusetts, USA/Amersterdam, Netherlands.
Gelman, A., J. B. Carlin, H. S. Stern, and D. R. Rubin. 1995. Bayesian data analysis. Chapman and Hall, New York, USA.
Gill, F. B. 1980. Historical aspects of hybridization between Blue-winged and Golden-winged Warblers. The Auk 97:1–18.
Gill, F. B. 2004. Blue-winged Warblers (Vermivora pinus) versus Golden-winged Warblers (V. chrysoptera). The Auk 121:1014–1018.
Johnson, R. R., C. K. Baxter, and M. E. Estey. 2008. An emerging agency-based approach to conserving populations through strategic habitat conservation. Pages 201–224 in J. J. Millspaugh and F. R. Thompson III, editors. Models for planning wildlife conservation in large landscapes. Academic/Elsevier, Boston, Massachusetts, USA/Amersterdam, Netherlands.
Kubel, J. E., and R. H. Yahner. 2007. Detection probability of Golden-winged Warblers during point counts with and without playback recordings. Journal of Field Ornithology 78:195–205.
Link, W. A., and J. R. Sauer. 2002. A hierarchical analysis of population change with application to Cerulean Warblers. Ecology 83:2832–2840.
Manel, S., M. K. Schwartz, G. Luikart, and P. Taberlet. 2003. Landscape genetics: combining landscape ecology and population genetics. Trends in Ecology and Evolution 18:189–197.
Martinez-Meyer E., A. T. Peterson, and W. W. Hargroves. 2004. Ecological niches as stable distributional constraints on mammal species, with implications for Pleistocene extinctions and climate change projections for biodiversity. Global Ecology and Biogeography 13:305–314.
Peterson, A. T., M. A. Ortega-Huerta, J. Bartley, V. Sanchez-Cordero, J. Soberón, R. H. Buddemeier, and D. R. B. Stockwell. 2002. Future projections for Mexican faunas under global climate change scenarios. Nature 416:626–629.
Peterson, A. T., and C. R. Robins. 2003. Using ecological-niche modeling to predict barred owl invasions with implications for spotted owl conservation. Conservation Biology 17:1161–1165.
Peterson, T. A., J. Soberón, and V. Sanchez-Cordero. 1999. Conservatism of ecological niches in evolutionary time. Science 285:1265–1267.
Rhymer, J. M., and D. Simberloff. 1996. Extinction by hybridization and introgression. Annual Review of Ecology and Systematics 27:83-109.
Rich, T. D., C. J. Beardmore, H. Berlanga, P. J. Blancher, M. S. W. Bradstreet, G. S. Butcher, D. W. Demarest, E. H. Dunn, W. C. Hunter, E. E. Iñigo-Elias, J. A. Kennedy, A. M. Martell, A. O. Panjabi, D. N. Pashley, K. V. Rosenberg, C. M. Rustay, J. S. Wendt, and T. C. Will. 2004. Partners in Flight North American Landbird Conservation Plan. Cornell Lab of Ornithology, Ithaca, NY, U.S.A.
Rissler, L. J., R. J. Hijmans, C. H. Graham, C. Moritz, and D. B. Wake. 2006. Phylogeographic lineages and species comparisons in conservation analysis: a case study of California herpetofauna. American Naturalist 167:655–666.
Scribner, K.T., J.A. Blanchong, D.J. Bruggeman, B.K. Epperson, C.-Y. Lee, Y.-W. Pan, R.I. Shorey, B. Williams, H.H. Prince, S.R. Winterstein, and D.R. Lukkonen. 2005. Geographical genetics: conceptual foundations and empirical applications of spatial genetic data in wildlife management. Journal of Wildlife Management 69:1434–1453.
Shapiro, L. H., R. A. Canterbury, D. M. Stover, and R. C. Fleischer. 2004. Reciprocal introgression between Golden-winged Warblers (Vermivora chrysoptera) and Blue-winged Warblers (V. pinus) in eastern North America. The Auk 121:1019-1030.
Spiegelhalter, D. J., A. Thomas, and N. G. Best. 2003. WinBUGS Version 1.4 user manual. Medical Research Council Biostatistics Unit, Cambridge, UK.
Stephens, P. R., and J. J. Wiens. 2009. Bridging the gap between community ecology and historical biogeography: niche conservatism and community structure in emydid turtles. Molecular Ecology 18:4664–4679.
Storfer, A., M. A. Murphy, J. S. Evans, C. S. Goldberg, S. Robinson, S. F. Spear, R. Dezzani, E. Delmelle, L. Vierling, and L. P. Waits. 2007. Putting the ‘landscape’ in landscape genetics. Heredity 98:128–142.
Thogmartin, W. E. Modeling and Mapping Golden-winged Warbler Abundance to Improve Regional Conservation. Avian Conservation and Ecology - Écologie et conservation des oiseaux A(B): C. [online] URL: http://www.ace-eco.org/volXX/issYY/artZZ/
Thogmartin, W. E., M. G. Knutson, and J. R. Sauer. 2006. Predicting regional patterns in rare grassland bird abundance with a hierarchical spatial count model. Condor 108:25–46.
Thogmartin, W. E., J. R. Sauer, and M. G. Knutson. 2004. A hierarchical spatial model of avian abundance with application to Cerulean Warblers. Ecological Applications 14:1766–1779.
Thogmartin, W. E., J. R. Sauer, and M. G. Knutson. 2007. Modeling and mapping abundance of American woodcock across their breeding range in the United States. Journal of Wildlife Management 71:376–382.
Vallender, R., V. L. Friesen and R. J. Robertson. 2007a. Paternity and performance of Golden-winged Warblers (Vermivora chrysoptera) and Golden-winged X Glue-winged Warbler (V. pinus) hybrids at the leading edge of a hybrid zone. Behavioral Ecology and Sociobiology 61:1797-1807.
Vallender, R., R. J. Robertson, V. L. Friesen, and I. J. Lovette. 2007b. Complex hybridization dynamics between Golden-winged and Blue-winged Warblers (Vermivora chrysoptera and V. pinus) revealed by AFLP, microsatellite, intron and mtDNA markers. Molecular Ecology 16: 2017-2029.
Vallender, R., S. L. Van Wilgenburg, L. P. Bulluck, A. Roth, R. Canterbury, J. Larkin, R. Fowlds, and I. J. Lovette. 2009. Extensive rangewide mitochondrial introgression indicates substantial cryptic hybridization in the Golden-winged Warbler (Vermivora chrysoptera). Avian Conservation and Ecology - Écologie et conservation des oiseaux 4(2): 4. [online] URL: http://www.ace-eco.org/vol4/iss2/art4/
Vogelmann, J. E., S. M. Howard, L. Yang, C. R. Larson, B. K. Wylie, and N. Van Driel. 2001. Completion of the 1990s National Land Cover Data set for the conterminous United States from Landsat Thematic Mapper data and ancillary data sources. Photogrammetric Engineering and Remote Sensing 67:650–652.
Wiens, J. J., C. H. Graham, D. S. Moen, S. A. Smith, and T. W. Reeder. 2006. Evolutionary and ecological causes of the latitudinal diversity gradient in Hylid frogs: Treefrog trees unearth the roots of high tropical diversity. American Naturalist 168:579–596.
Will, T. C. 1986. The behavioral ecology of species replacement: Blue-winged and Golden-winged Warblers in Michigan. Dissertation. University of Michigan, Ann Arbor, Michigan, USA.
Zhu, Z. and D. L. Evans. 1994. U.S. forest types and predicted percent forest cover from AVHRR Data. Photogrammetric Engineering and Remote Sensing 60:525–531.
Table 1. The Minnesota population of Golden-winged Warblers exhibited lower observed levels of introgression and overlap with Blue-winged Warblers compared to the Wisconsin population.
|
State |
|
Scale of Interaction |
MN |
WI |
Extent of Introgression (%)a |
1.04 |
9.76 |
Mean Ratio of Overlap |
4.44 |
8.37 |
a As reported by Vallender et al. (2009)
Figure Legend
Figure 1. Predicted patterns of relative abundance for Golden-winged Warblers (a) and Blue-winged Warblers (b) in Bird Conservation Region 23, the Prairie Hardwood Transition. Cities (in black) are mapped to provide additional spatial reference.
Figure 2. Predicted patterns in relative extent of overlap for the Golden-winged Warbler and Blue-winged Warbler in the Prairie Hardwood Transition of the upper Midwestern United States. The inset identifies patterns in the abundance of the Golden-winged Warbler relative to areas of predicted overlap.
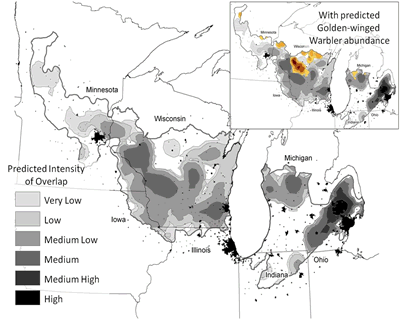
URL: http://umesc.usgs.gov/terrestrial/migratory_birds/species_distributional_modeling.html
Page Contact Information: Contacting the Upper Midwest Environmental Sciences Center
Page Last Modified: February 1, 2016